Volatilomic profiling reveals altered wild-type Pseudmonas aeruginosa metabolism compared to quorum sensing loss-of-function mutant strains
Waqar Ahmed, Taoran Fu, Kamila Schmidt, Michael Brockhurst, Stephen J Fowler
University of Manchester, UK
Once a minimum cell density threshold is reached, Pseudomonas aeruginosa excretes small signaling compounds which activate functional genes responsible for virulence mechanisms. In this way, P. aeruginosa can persist in biofilms, form synergistic or parasitic interactions with commensal microbes, or invade a host causing life-threatening systemic infection1. In this pilot study we use gas chromatography-mass spectrometry to measure volatile metabolites from wild-type and loss-of-function mutant strains lacking in quorum sensing (QS) systems to uncover differences in cellular metabolism associated with QS signaling. Our initial results indicate 2-aminoacetophenone (2-AA) in culture headspace is lower in abundance for wild-type strains compared to lasR and rhl loss-of-function strains. This demonstrates that production of 2-AA, a metabolite of the PQS system is affected by the function of lasR and rhl and therefore confirms P. aeruginosa QS systems are interlinked and inter-dependent. This may have implications in breath biomarker discovery for infection2 as microbial metabolites have varied concentrations which are potentially dependent on intracellular interactions amongst other factors such as host response, nutrient availability, and cell concentration.
1Smith RS, Iglewski BH. P. aeruginosa quorum-sensing systems and virulence. Current Opinions in Microbiology 2003, 6(1), 56-60
2Ahmed WM, Fenn D, White IR, Dixon B, Nijsen TME, Knobel HH, Brinkman P, Van Oort PMP, Schultz MJ, Dark P, Goodacre R, Felton T, Bos LDJ, Fowler SJ, BreathDx Consortium. Microbial volatiles as diagnostic biomarkers of bacterial lung infection in mechanically ventilated patients. Clinical Infectious Diseases 2023, 76(6), 1059-1066.
The authors declare that they have no conflict of interest.
|
Developing a Breath Test for Valley Fever using GC×GC Untargeted Volatilomics
Emily A Higgins Keppler1,2, Heather L Mead3, Marley C Van Dyke4, Douglas F Lake1, D Mitch Magee5, Bridget M Barker6, Heather D Bean1,2
1School of Life Sciences, Arizona State University, Tempe, AZ
2Center for Fundamental and Applied Microbiomics, The Biodesign Institute, Tempe, AZ
3The Translational Genomics Research Institute (TGen), Phoenix and Flagstaff, AZ
4Microbiology Department, UT Southwestern Dallas, TX
5 Center for Personalized Diagnostics, Biodesign Institute, Arizona State University, Tempe, AZ
6 The Pathogen and Microbiome Institute, Northern Arizona University, Flagstaff, AZ
Background
Valley fever (coccidioidomycosis) is an endemic fungal pneumonia of the arid regions of North and South America. It is estimated there are 350,000 new cases of Valley fever each year, and in endemic and highly populated regions (e.g., Phoenix, Arizona and the San Joaquin Valley of California) up to 30% of community-acquired pneumonia (CAP) may be caused by Valley fever. The current diagnostics for Valley fever are severely lacking due to poor sensitivity and specificity, especially early in infection, which leads to delayed diagnosis, inappropriate treatment with antibiotics, lost productivity, and increased medical costs.
Objective
We are working toward the development of a breath test to discriminate Valley fever from other causes of CAP. In this study we performed untargeted volatilomics analyses of bronchoalveolar lavage fluid (BALF) samples from murine Coccidioides lung infections and BALF from persons with CAP to identify putative volatile biomarkers of Valley fever.
Methods
Murine model: All procedures were approved by the Institutional Animal Care and Use Committee (protocol 16–011) of Northern Arizona University. Three cohorts of mice were infected by intranasal inoculation with C. posadasii Silveira (n=6), C. immitis RS (n=6), or vehicle control (n = 4). After 10 days of infection, the mice were euthanized and 2 mL of BALF was collected for volatile metabolomics analyses and cytokine analysis by a mouse magnetic 26-Plex ProcartaPlexTM panel. Human samples: Mayo Clinic Arizona provided 55 BALF specimens, divided into three categories: coccidioidomycosis (n=14), non-Coccidioides CAP (n=29), and uninfected (n=12). All volatilomics samples were divided into technical triplicates and analyzed by headspace solid phase microextraction (SPME) and two-dimensional gas chromatography coupled with time-of-flight mass spectrometry (GC×GC-TOFMS). Random forest was performed on the human volatilomes to identify putative biomarkers to discriminate Valley fever from other causes of CAP.
Results
We detected 244 VOCs in the human BALF samples, eight of which could distinguish Coccidioides pneumonia from non-Cocci infected samples, and specifically from bacterial pneumonia, with > 95% accuracy. Data from the murine model suggest that a significant portion of the Valley fever volatiles are produced by the host and correlated with the immune response.
Conclusion
Combined, these pilot data indicate that a breath test to discriminate Valley fever from other causes of CAP is feasible and may facilitate antimicrobial stewardship through improved fungal detection and the stratification of disease severity.
Acknowledgements and Disclosures
This study was supported by an Arizona Biomedical Research Centre New Investigator Award to H.D.B. There are no conflicts of interest or financial disclosures for this study.
|
Are we throwing the biomarkers out with the bath water?
Scott Borden, Kristian Kiland, Crista Bartolomeu, Lucas Martins, Stephen Lam, Renelle Myers
Integrative Oncology, BC Cancer Research Centre, Vancouver, Canada
Background
Universal data pre-processing strategies that account for the influence of room air during breath biomarker discovery have yet to be established. Meticulous care must be used to account for the VOCs in room air that a participant inhales prior to breath collection, as well as background exogenous environmental VOCs. Data preprocessing strategies to address this have consequently been aggressive to avoid false discovery, removing 100s of features. Accepted methods include requiring the breath signal to be larger than the room air signal or larger than the mean + 3× standard deviation. Other alternatives use a 3 µg/m3 threshold. Complicating this matter is the breath collection method used. Modern breath collection devices like the ReCIVAä differ significantly from one-breath exhalation devices as they involve the patient breathing in highly purified air for several breaths prior to starting, and throughout the collection. The sample is collected from an aggregate of multiple expirations over 5-10 minutes. Data processing has not been adapted for these devices.
Objective
To investigate the influence of exogenous environmental VOCs on breath samples acquired via the ReCIVAä device (breathing purified air).
Methods
Two healthy participants gave baseline breath samples, then were exposed to a selected VOC mixture for 30 minutes prior and during breath collection via ReCIVAä. Serial breath collections were then taken at 10, 20, 30, 60, and 120 minutes post exposure, in a clean room and compared for 12 target analytes. Peppermint, lemongrass, and cinnamon essential oils (EO) were prepared at 20 ppm in methanol and analyzed by TD-GC-MS to identify 12 target analytes. The EO mixture was dispersed in a small room (115 ft2) for one hour using a household diffuser prior to participant entering. All breath and room air samples were collected (1000 cm3) onto thermal desorption tubes and analyzed by thermal desorption gas chromatography mass spectrometry (TD-GC-MS).
Results
A total of 12 VOCs from the EOs were screened to assess the peak area response at each time point relative to the room air sample from the EO room. Room air samples from the EO room yielded large signals, with S/N values ranging from 2.8×105 to 2.2×106. Nine of twelve screened VOCs were not able to be detected in any ReCIVAä breath data. The stark difference in signal is illustrated in Figure 1, demonstrating the room air peaks for neral, citral, and eugenol relative to the breath sample taken during EO exposure. Despite the large room air signals observed, nine of these twelve analytes were unable to be detected in ReCIVAä breath samples at any time point. Three of twelve analytes showed minor signals (<2.5% of signal relative to room air) in the exhaled breath sample taken in the EO room; these VOCs were those that arose from the peppermint EO and are often observed in breath.
Conclusions
Advances in breath sampling devices have greatly attenuated the influence of the environment on patient breath samples. Common preprocessing strategies currently employed may be overestimating the influence of room air and consequently removing relevant features.
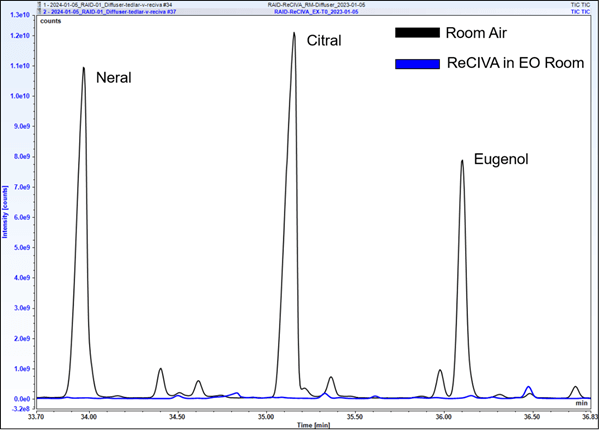
Figure 1. Comparison of neral, citral, and eugenol peaks in room air, and from a ReCIVAä exhaled breath sample taken in the EO VOC-rich room. Additional time points are not shown for visual clarity, as they are also below detection limits
|
Building a Metabolomic Database for Comprehensive Analysis of Exhaled Breath Composition and Biomarker Identification
Eva Borras, Mitchell M McCartney, Cristina E. Davis
Mechanical and Aerospace Engineering, University of California, Davis, Davis, CA
Background
Exhaled breath condensate (EBC) is a matrix with particular interest for being a non-invasive and easily accessible biospecimen that can be collected without the need for complex procedures. EBC also contains valuable information of the composition of the respiratory system. Biomarker discovery and changes in metabolomic composition in EBC may indicate the presence of respiratory diseases such as asthma, chronic obstructive pulmonary disease (COPD), or even systemic conditions like diabetes.
While targeted approaches allow quantification of known compounds associated to specific diseases, they are focused on a predefined set of compounds. This can limit the coverage of the metabolome, overlooking unexpected metabolites that could be biologically relevant. Nevertheless, untargeted metabolomic approaches allow a broad and comprehensive profiling of the entire breath metabolome, enabling the discovery of novel biomarkers for respiratory or systemic conditions, and facilitating the exploration of entire metabolic pathways. The combination of both approaches can provide a better understanding of the metabolome, however, there are some challenges associated to untargeted liquid chromatography-mass spectrometry (LC-MS) metabolomics. One of the main limitations is the confidence in metabolite identifications, matrix effects, isomeric co-elutions or quantification of the new biomarkers, needing spectral databases or authentic standards for verifications.
Moreover, in the case of EBC, the sample amount is limited and often requires intense pre-concentration steps to detect metabolites. Analytical quality control practices are usually challenging when working with this matrix, and the re-analyzing of samples for a proper characterization (e.g. MSn) is often not possible. The use of authentic standards can enhance the analytical method, use as quality control, serve as reference compounds for validation, and index retention times by creating spectral libraries by commercial standards databases. These libraries contain mass spectra and retention time information for a wide range of metabolites that can be compared with experimental spectra from samples and allow better putative identifications. Additionally, these compounds can be quantified and asses concentration changes in EBC.
In this study, we present the development of a metabolomic database using commercially available metabolite libraries to enhance our understanding of the EBC composition and the identification of potential new biomarkers.
Methods
We compiled a metabolomic database by integrating four commercially available metabolite libraries, ensuring coverage across a wide range of metabolite classes. This comprehensive database, containing over 1500 metabolites, serves as a reference library for the identification and quantification of metabolites present over 400 EBC samples from different studies. LC-MS was employed for high-throughput profiling of EBC samples, with subsequent data processing and annotation using the established metabolomic database.
Results
The new metabolomic database includes a diverse array of known metabolites, including volatile organic compounds, lipids, amino acids, and small molecules from 400 samples. Analysis of EBC samples from different cohorts revealed a complex and dynamic metabolomic profile. Preliminary findings indicate significant variations in the abundance of specific metabolites associated with asthma, influenza, COVID-19, or even effects of flu vaccination of exposure of wildfires. These results can contribute to our better understanding of metabolic pathways implicated in respiratory and systemic health.
|
ONELAB: applications of breath volatile analysis to rapidly screen for pathogenic infections
Anesu Chawaguta1, Paul Brinkman2, Zoltan Gyongyi3, Chris A. Mayhew1, Veronika Ruzsanyi1, Daniel Sanders4, Makoto Sawano5, Matthias Schlögl6 and C. L. Paul Thomas7
1Institute for Breath Research, Universität Innsbruck, Innsbruck, Austria
2Department of Respiratory Medicine, Amsterdam UMC, University of Amsterdam, Amsterdam, Netherlands
3University of Pec, Pecs, Hungary
4G.A.S. Gesellschaft für Analytichesensensorsysteme GmbH, Dortmund, Germany
5Saitama Medical University, Saitama, Japan
6Solgeium OG, Linz, Austria
7Bioxhale Ltd, Leicester, United Kingdom
Background
The outbreak of the COVID-19 pandemic showed how unprepared and uncoordinated governments were to the entry of a novel virus into the human population. A major problem was the lack of a rapid response to mitigate the impact of the SARS-CoV-2 virus on society. To help lessen the potential impact of future emergent viral infections, a recently funded Horizon Europe Programme and UK Research and Innovation project called “ONELAB” is developing next-generation modular rapid response mobile laboratories for flexible, scalable and multi scenario deployments of analytical devices and clinical tests to provide point-of-need tests to determine infections with a high-level of situational awareness [1].
Objective
A key objective of ONELAB is to screen people rapidly and accurately for pathogenic infection at the outbreak of a disease when much is still undefined. An aim is to provide rapid triage to determine a person’s infection status. A key element to this diagnostic capability is the use of acute volatile infection biomarkers present in exhaled breath that can be used to determine whether a contagion is present or not. A proof-of-principle study has already demonstrated the potential of using breath volatiles to diagnose SARS-CoV-2 infection [2]. ONELAB significantly expands on this earlier seminal work by undertaking longitudinal observational epidemiological studies over two winters to identify and monitor volatiles in exhaled breath that are associated with acute respiratory tract infections, involving four clinical partners based in Europe and Japan. Using Gas Chromatography Mass Spectrometry (GC-MS) for volatile discovery and GC-Ion Mobility Spectrometry (GC-IMS) for near-to-real time breath pattern profiling, we will acquire the knowledge to develop GC-IMS as a technological platform for use in a non-invasive breath infection-screening breath test as a first line of defence procedure to protect people, healthcare systems, and economies.
Discussion
In January 2024, ONELAB started to obtain breath samples for analysis from infected patients and from controls from all four clinics, with a target of 1000 samples to be collected and analysed, making this the largest breath pathogenic infection study to date. The GC-IMS pathogenic volatile breath fingerprints are being incorporated into innovative machine-learning pattern recognition algorithms to profile the signature patterns of infection in the breath volatilome for direct use in screening. Preliminary GC-MS and GC-IMS breath sample analyses will be presented together with our initial outcomes from the machine learning algorithms.
Acknowledgement
We acknowledge the EU HORIZON Innovation Actions HORIZON-CL3-2021-DRS-01-05, Project Number 101073924 (ONELAB) for funding this work.
References
[1] https://onelab-project.eu/
[2] D. Ruszkiewicz et al eClinicalMedicine 29-30 (2020) doi: 100609 doi: 10.1016/j.eclinm. 2020.100609
|
Assessment of Exhaled Breath VOC Stability on Tenax® GR Thermal Desorption Tubes
Trenton J. Davis1,2, Ivan V. Ivanov3, Darakshan Zabin1, Heather D. Bean1,2
1School of Life Sciences, Arizona State University, Tempe, AZ
2Center for Fundamental and Applied Microbiomics, The Biodesign Institute, Tempe, AZ
3Department of Veterinary Physiology & Pharmacology, Texas A&M University, College Station, TX
Background
The analysis of exhaled breath volatile organic compounds (VOCs) is increasingly being leveraged to study human health and disease including respiratory infections, asthma, and metabolic disorders. Sampling of breath VOCs often involves transferring breath samples onto sorbent-packed thermal desorption tubes (TDTs) for concentration, transport, and storage. Due to constraints such as distant sampling locations and instrument capacity, samples cannot always be same-day analyzed and must be stored for a future processing date, and sometimes must be shipped using air or ground transportation. This brings forth questions regarding the most appropriate storage method, specifically with regards to storage length, for maintaining sample integrity.
Objective/Aims
The goal of this study was to examine the effects of storage time at 4 °C and shipping method (air vs. ground shipping) on the recovery of exhaled breath VOCs from Tenax® GR TDTs.
Methods
All procedures were approved by the U.S. Army Medical Research and Development Command (USAMRDC), Office of Human and Animal Research Oversight (OHARO), and Office of Human Research Oversight (OHRO; log numbers E03915.1a and E03915.1a-1). Three experiments were conducted: Experiment 1 tested the variable of storage time alone; Experiment 2 tested storage time plus air shipping vs. no shipping; and Experiment 3 tested storage time plus ground shipping vs. air shipping vs. no shipping. For each experiment, breath was collected in 10 L Tedlar bags from four subjects and split onto 12 to 14 technical replicate Tenax® GR TDTs. Exp 1 samples were stored at 4 °C and technical duplicates were analyzed using thermal desorption – comprehensive two-dimensional gas chromatography – time of flight mass spectrometry (TD–GC´GC–TOFMS) after 0.5, 1, 2, 4, 8, 16, and 32 days; Exp 2 technical duplicates were analyzed after 8, 16, and 32 days; Exp 3 technical duplicates were analyzed after 16 and 32 days.
Results
We found no significant effect of storage time for up to 32 days on the relative abundances and the numbers of detected VOCs, or on the reproducibility of the breath VOCs on technical duplicate samples. Similar results were observed when air and ground shipping were taken into consideration.
Conclusions
Overall, these results demonstrate that exhaled breath VOCs collected and shipped under these conditions can be stored for upwards of a month without jeopardizing sample integrity.
Acknowledgements and Disclosures
This work was supported by the Defense Advanced Research Projects Agency (DARPA) Fatigue Assessment via Breath (FAB) study (Cooperative Agreement HR00112220040; Roozbeh Jafari and Linda Katehi, PIs). The views, opinions, and/or findings contained in this material are those of the authors and should not be interpreted as representing the official views or policies of the Department of Defense or the U.S. Government. No official endorsement should be inferred. There are no conflicts of interest or financial disclosures for this study.
|
Quality Control Framework for Exhaled Breath VOC Analaysis Using Thermal Desorption
Trenton J. Davis1,2, Bilal N. Ali1, Darakshan Zabin1, Heather D. Bean1,2
1School of Life Sciences, Arizona State University, Tempe, AZ
2Center for Fundamental and Applied Microbiomics, The Biodesign Institute, Tempe, AZ
Background
Sampling exhaled breath volatile organic compounds (VOCs) for offline analysis often involves multiple steps, including the collection of exhaled breath in bags, transferring the breath via a pump to sorbent-packed thermal desorption tubes (TDTs) onto which the VOCs adsorb, storing and transporting samples to the analytical lab, and then desorbing the VOCs from the TDTs into the inlet of a gas chromatograph. Due to the complexities and expense of human sampling, exhaled breath samples are precious, and great care is undertaken to maintain and assess their integrity through the sampling and storage/handling process. These efforts are not always followed through to analysis, however, and many researchers fail to adequately evaluate analysis quality (e.g., sufficient sample desorption and injection). Taking from process engineering, we present an easily implemented statistical quality control framework for post-hoc monitoring of breath sample analysis via thermal desorption – gas chromatography (TD–GC).
Methods
All procedures were approved by the U.S. Army Medical Research and Development Command (USAMRDC), Office of Human and Animal Research Oversight (OHARO), and Office of Human Research Oversight (OHRO; log numbers E03915.1a and E03915.1a-1). Four subjects were recruited to provide three breath samples in 10 L Tedlar bags. All breath samples were collected on the same day, and within 120 minutes of sample collection, each sample was split into 0.6 L technical replicates by transferring onto Tenax® GR TDTs. TDTs (n = 152) were stored at 4 °C and technical duplicate TDTs were analyzed after 0.5 to 32 days of storage using thermal desorption – comprehensive two-dimensional gas chromatography – time of flight mass spectrometry (TD–GC´GC–TOFMS). Multivariate T2 single control charts of five low-variance VOCs detected across all samples were calculated using the R package “qcc”. Out-of-control (OOC) samples were defined as those with T2 statistics exceeding (1–0.0027)p, where p is the number of variables and 0.0027 is the probability of a Type I error for a single Shewhart chart at the mean ± 3 (s.d.).
Results
Using the multivariate Hoteling’s T2 statistic of five endogenous breath VOCs, we identified OOC breath samples at a rate not greater than 5% in an analysis of 152 breath TDT samples.
Conclusions
We show that this quality control framework can quickly identify OOC breath samples post-analysis, enabling the exclusion of the samples from downstream data processing, or the replacement of the OOC sample with a back-up technical replicate in order to preserve the sample size of the study.
Acknowledgements and Disclosures
This work was supported by the Defense Advanced Research Projects Agency (DARPA) Fatigue Assessment via Breath (FAB) study (Cooperative Agreement HR00112220040; Roozbeh Jafari and Linda Katehi, PIs). The views, opinions, and/or findings contained in this material are those of the authors and should not be interpreted as representing the official views or policies of the Department of Defense or the U.S. Government. No official endorsement should be inferred. There are no conflicts of interest or financial disclosures for this study.
|
Quantitative analysis of a broad range of carbonyl compounds in exhaled breath for detection of COVID-19 during the periods of SARS-CoV-2 Alpha and Delta variants
Zhenzhen Xie; James Morris1; Jianmin Pan; Elizabeth A. Cooke; Saurin R. Sutaria; Dawn Balcom; Subathra Marimuthu; Leslie W. Parrish; Holly Aliesky;Justin J. Huang; Shesh N. Rai; Forest W. Arnold; Jiapeng Huang; Michael Nantz; Xiao-an Fu
University of Louisville
Quantitative analysis of a broad range of carbonyl compounds in exhaled breath for detection of COVID-19 during the periods of SARS-CoV-2 Alpha and Delta variants Background: COVID-19 has caused a worldwide pandemic, creating an urgent need for early detection methods. Breath analysis has shown great potential as a non-invasive and rapid means for COVID-19 detection. Volatile organic compounds (VOCs) are generated by host responses through a series of lipid degradations including ketosis and inflammatory processes present in the lungs. Breath analysis techniques also demonstrated the potential for differentiation of variants of SARS-Cov-2. Many variants of SARS-Cov-2 create challenges for its detection and curbing the disease.
Objective
The objective of this study is to detect COVID-19, differentiate of Alpha from the Delta variant, and detect asymptomatic COVID-19 infection by analysis of carbonyl compounds in exhaled breath using a microreactor approach.
Methods
The study included a cohort of COVID-19 positive and negative subjects confirmed by reverse transcriptase polymerase chain reaction between March and December 2021. Carbonyl compounds in exhaled breath were captured using a microfabricated silicon microreactor and analyzed by ultra-high performance liquid chromatography-mass spectrometry (UHPLC-MS). A total of 341 subjects were enrolled in this study. Of these, 141 (85 males, 60.3%) (mean±SD age: 51.6±15.1 years) were COVID-19 positive and 180 (90 males, 50%) (mean±SD age: 45.2±14.9 years) were negative. Panels of ketones and aldehydes in breath samples were identified for detection of COVID-19 positive patients. Logistic regression models were employed for the data. The model performance was evaluated by the ROC curve with AUC, accuracy, sensitivity and specificity.
Results
Logistic regression models indicated high accuracy/sensitivity/specificity for alpha variant (98.4%/96.4%/100%), for delta variant (88.3%/93.0%/84.6%), for all COVID-19 positive patients (94.7%/90.1%/98.3%), and for asymptomatic patients (95.3%/71.0%/99.5%).
Conclusion
COVID-19 positive patients can be detected by analysis of carbonyl compounds in exhaled breath by microreactor approach. The technology for analysis of carbonyl compounds in exhaled breath has great potential for rapid screening and detection of COVID-19 and for other infectious respiratory diseases in the future endemics.
|
Assessing response to phage therapy of M. abscessus using breath
Antao Gao1, Jerry A. Nick2,3, Rebekah M. Dedrick4, Graham F. Hatfull4, Katie Poch2, Silvia Caceres2, Ahmad Mani1, Jane E. Hill1
1Department of Chemical and Biological Engineering, University of British Columbia, Vancouver, BC V6T 1Z4, CA
2Department of Medicine, National Jewish Health, Denver, CO 80206, USA
3Department of Medicine, University of Colorado School of Medicine, Aurora, CO 80045, USA
4Department of Biological Sciences, University of Pittsburgh, Pittsburgh, PA 15260, USA
Background
Mycobacterium abscessus (M.abs) is a nontuberculous mycobacteria (NTM) that may cause severe lung infection in people with cystic fibrosis (pwCF). High levels of intrinsic and acquired antibiotic resistance in M. abs result in limited treatment options and often lead to multiple relapses and low cure rates. This may prevent pwCF from receiving lung transplant. Phages are viruses that attack bacteria selectively, and their therapeutic use has been evaluated for difficult-to-treat infections, with recent successful phage treatments of M. abs in pwCF. The sensitivity of NTM microbial culture in cystic fibrosis airways can be as low as 30%. Thus, there is a growing need for better culture-independent markers of treatment response. Exhaled breath, capturing volatile compounds emitted by bacteria or the host, is a promising candidate for such biomarkers.
Hypotheses
1) Volatile biomarkers in breath can differentiate between M. abs positive and M. abs negative cases; and 2) Phage therapy for M. abs can be reflected in the variation of the abundance of volatile biomarkers in breath.
Methods
Two engineered phages were used to treat M. abs infection in an individual with advanced cystic fibrosis (CF) lung disease. A variety of markers combined with extensive cultures indicated eradication of M. abs following a year of treatment, allowing for successful lung transplant. Breath samples (3 Liter) were collected at multiple time points prior to the phage therapy till after the lung transplant operation (Figure 1). Breath samples were collected into Tedlar bags and concentrated onto thermal desorption tubes (TDTs) which were then analyzed using two-dimensional gas chromatography coupled with a time-of-flight mass spectrometry (GC×GC ToFMS). Chemical compounds were identified by spectral library match, and putative diagnostic features were selected by statistical analysis using R programming.
Results: Fourteen volatile compounds in breath were identified that differentiated between M. abs positive (M. abs+, n=7) and M. abs negative (M. abs-, n=4) breath samples (Figure 2a). The changes in the relative abundances of a subset of these 14 volatile compounds were consistent with variations in other biomarkers, including microbial culture, immunoglobulin (IgA and IgG), urine lipoarabinomannan (LAM), and M.abs DNA (Figure 2b). Five out of the 14 volatile compounds were detected in breath samples (n=8) collected after lung transplant, and these five biomarkers correctly classified all the breath samples as M. abs-, which conformed to the culture results (Figure 2c and 2d). Chemical identity of three compounds were confirmed by authentic chemical standards.
Conclusion
There are 14 compounds in breath of this patient that could diagnose M. abs lung infection and correlate to phage treatment efficacy during an M.abs lung infection in a pwCF.
The study protocol was approved by the University of British Columbia Research Ethics Boards. This study was supported by the US National Institutes of Health, R01 HL146228-01. The authors declare no conflict of interest.
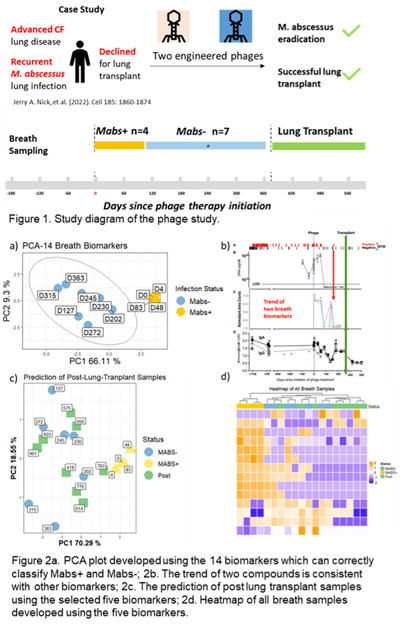
|
Analysis of volatile aldehydes using secondary electrospray ionization mass spectrometry.
S. Giannoukos1, E. Lattouf 1, M. Emery1, R. Zenobi1
1 Department of Chemistry and Applied Biosciences, ETHZ, Zurich, Switzerland
Background
Aliphatic straight-chain aldehydes have been consistently identified in the breath of individuals with lung diseases through multiple detection methods, including mass spectrometry, ion mobility spectrometry, and electrochemical sensors. Research has revealed elevated levels of these exhaled aldehydes in patients with various lung conditions, including lung cancer, inflammatory and infectious lung diseases, as well as mechanical lung injuries. Quantifying straight-chain aliphatic aldehydes in the clinical setting is thus highly important.
Objective/Aims
This work focuses on the qualitative detection and quantification of volatile straight-chain aliphatic aldehydes using an online secondary electrospray ionization source coupled to a high-resolution mass spectrometry system.
Methods
A secondary electrospray ionization (SESI) source coupled to a high-resolution mass spectrometry (HRMS) system was employed for the first time to detect and monitor, both qualitatively and quantitatively, selected volatile straight-chain aliphatic aldehydes in the gaseous phase. The generation of gas standards was done using a built-in-house vapor generator based on the controlled evaporation of volatile or semi-volatile chemical analytes and their diffusion into a carrier gas stream. The main components of the vapor generator are a) a mixing chamber, b) three individual temperature-controlled evaporation chambers in which liquid analytes are introduced through a side injection port, c) four mass flow controllers, and d) an automation platform controlled by software. SESI-HRMS is a powerful, well-established, and robust analytical technique ideal for in-depth breath metabolomics characterization, offering high sensitivity (low limits of detection), selectivity, fast (within seconds), and accurate analysis.
Results
Experiments were undertaken for propanal, butanal, pentanal, hexanal, heptanal, octanal, nonanal, and decanal at different concentrations and flow rates. Both individual compounds and mixtures were tested. Gas-phase experiments were performed at concentration levels from low ppt to low ppm and in both dry and humid conditions. The experimental results obtained showed a precise and repeatable production of gas standards with excellent linearity within the examined concentration range, low ppt detection limits, and fast response times.
Conclusions
For the first time, the qualitative and quantitative analysis of straight-chain aliphatic aldehydes was demonstrated using a dynamic vapor generator, capable of producing gaseous standards in a precise and reproducible way, with a SESI source coupled to a high-resolution mass spectrometer. The analytical performance of this approach was investigated by addressing essential analytical criteria such as limits of detection, limits of quantification of individual compounds, and the response in multi-component mixtures under dry and humid conditions in the full mass range m/z from 50 to 500.
|
Identifying putative volatile biomarkers for S. aureus methicillin-resistant and small-colony variant subtypes
Daniela F. Gutiérrez-Muñoz1,2, Daniel J. Wolter,3 Lucas R. Hoffman,3 Brandie D. Wagner,4 Heather D. Bean1,2
1School of Life Sciences, Arizona State University, Tempe, AZ
2Center for Fundamental and Applied Microbiomics, The Biodesign Institute, Tempe, AZ
3Department of Pediatrics, University of Washington, Seattle, WA
4Biostatistics & Informatics, Colorado School of Public Health, University of Colorado, Aurora, CO
Background
Seventy percent of persons with cystic fibrosis (pwCF) in the US are respiratory culture positive for Staphylococcus aureus, and this rate is climbing (1). With increasing S. aureus infections, the detection of clinically-relevant subtypes, such as methicillin-resistant S. aureus (MRSA) and small-colony variants (SCVs), is also increasing. Both subtypes, but especially thymidine-dependent SCVs, have been associated with worse patient outcomes (2, 3). Due to the high rates and co-occurrence of SCVs and MRSA (2), detecting and tracking the emergence of each of these S. aureus subtypes in CF lung infections is important for understanding the progression of CF lung disease and for formulating effective treatment options.
Objective/Aims
We are working to develop breath tests for detecting S. aureus MRSA and SCV lung infection subtypes in situ. As a first step in this process, we have characterized the in vitro volatile organic compounds (VOCs) produced by 110 S. aureus isolates from CF lung infections, representing four classes: methicillin-sensitive S. aureus normal colony variants (MSSA-NCVs), MSSA-SCVs, MRSA-NCVs and MRSA-SCVs.
Methods
The S. aureus clinical isolates in this study originated from pwCF and were phenotyped for methicillin sensitivity and SCV thymidine auxotrophy. The S. aureus cultures were resuspended from glycerol stocks in 100 ml LB-Lennox and plated as a lawn onto LB-Lennox agar supplemented with 3 mM glucose and 50 mg/ml thymidine, then cultured at 37°C for 24 h. The headspace VOCs of the cultures were sampled for 4 h at 37°C using thin-film microextraction then analyzed using comprehensive two-dimensional gas chromatography and time-of-flight mass spectrometry. VOCs associated with MRSA, SCVs, and MRSA-SCVs will be identified using random forest classification models.
Results
We observed that the random forest classification model performed best when comparing the MSSA-NCV subtype against the MRSA-SCV subtype, with an out-of-bag (OOB) error of 23.7%. Conversely, the model comparing the MSSA-SCV subtype against the MRSA-NCV subtype calculated a 41.7% OOB error. The VOCs of the MRSA-SCV subtypes seem to be unique and driving the classification models.
Conclusions
The next steps of this study will be to test the sensitivity and specificity of the VOC biomarkers we identify using independent isolates and to determine whether the discriminatory VOCs are detected in the breath of pwCF who have S. aureus lung infections.
Acknowledgements
This work was supported by the National Institutes of Health [R01HL157239] and bacterial isolates were obtained from the Seattle Children’s Center for CF Microbiology Isolate Core, funded by the NIH [P30 DK089507] and the Cystic Fibrosis Foundation [HOFFMA20Y2-OUT].
References
1. Cystic Fibrosis Foundation. Patient Registry Annual Data Report. Bethesda, MD: Cystic Fibrosis Foundation; 2021.
2. Wolter DJ, Onchiri FM, Emerson J, Precit MR, Lee M, McNamara S, et al. Prevalence and clinical associations of Staphylococcus aureus small-colony variant respiratory infection in children with cystic fibrosis (SCVSA): a multicentre, observational study. The Lancet Respiratory medicine. 2019;7(12):1027-38.
3. Dasenbrook EC, Checkley W, Merlo CA, Konstan MW, Lechtzin N, Boyle MP. Association Between Respiratory Tract Methicillin-Resistant Staphylococcus aureus and Survival in Cystic Fibrosis. JAMA. 2010;303(23):2386-92.
|
Determining Volatile Organic Compound Baselines in Healthy Breath with Tin Oxide Sensors: A Framework for Non-Invasive Health Monitoring
Shivaum Heranjal1,2, Mariana Maciel1, Sai Nishith Reddy Kamalapally1,3, Ishan Ramrakhiani1, Eray Schulz14, Dipak Maity1,4, Sha Cao5, Xiaowen Liu6, Ryan F. Relich7, Mark Woollam1,4, Mangilal Agarwal1,2,4,8
1Integrated Nanosystems Development Institute, Indiana University Indianapolis, IN, United States.
2Electrical and Computer Engineering, Purdue University Indianapolis, IN, United States.
3Mechanical and Energy Engineering, Purdue University Indianapolis, IN, United States.
4Chemistry and Chemical Biology, Indiana University Indianapolis, IN, United States.
5Biostatistics and Health Data Science, Indiana University School of Medicine, Indianapolis, IN, United States.
6Deming Department of Medicine, Tulane University School of Medicine, New Orleans, LA, United States.
7Pathology and Laboratory Medicine, Indiana University School of Medicine, Indianapolis, IN, United States.
8Biomedical Engineering and BioHealth Informatics, Luddy School of Informatics, Indiana University Indianapolis, IN, United States.
Background
Volatile organic compounds (VOCs) in breath serve as a rich source of biomarkers for many different medical conditions including cancer, infectious diseases, metabolic disorders, and other disease states. Electronic noses (e-Noses) are integrated arrays of gas sensors that rapidly respond to VOCs in exhaled breath which are cost-effective and portable devices. Even though e-Noses have been utilized for detecting an array of medical conditions through pattern recognition, an existing challenge is that many studies analyze VOC profiles without any prior knowledge of baseline response within healthy volunteers or how different confounding factors have an impact on sensor data.
Objective
The study sought to qualify healthy breath baselines of exhaled VOC profiles through analysis using an array of metal oxide (MOX) sensors.
Methods
Subjects were recruited/consented through word of mouth and using posters. For each sample, breath was analyzed using an array of MOX sensors with parameters that were previously optimized. To assess general health, data was also collected using a blood test and a lifestyle questionnaire. Sensor data was processed using a feature extraction algorithm, which was subsequently analyzed through statistical approaches to identify correlations with confounding factors. Reproducibility was also evaluated by examining the relative standard deviation values of sensor features both within an individual subject (longitudinal) and across various volunteers (cross-sectional).
Results
164 cross-sectional breath samples were collected from different individuals, and 10 of these volunteers provided an additional 9 samples over the course of 6 months for the longitudinal study. First, data from different subjects was analyzed, where 17 features were extracted from each of the sensor response curves and their trends were visualized. This showed a high degree of correlation between sensors within the array and even between some of the features extracted from the same sensor. This led to the removal of multicollinear features for multivariate statistical analyses. Confounding variables (biological sex, body mass index, smoking, and age) had an insignificant impact on the observed sensor signal as no correlations were identified between sensor features and confounding variables after p-value adjustment. Finally, the longitudinal samples were analyzed, which showed that the variability among individuals were notably greater compared to the variations observed within replicates of a single volunteer (p-value = .002). Analysis of the longitudinal data using multivariate methods indicated an inability to differentiate between subjects, indicating that there may be a universal healthy breath baseline that is not specific to individuals.
Conclusion
The current study sought to qualify healthy baselines of VOCs in exhaled breath using a MOX sensor array that can be leveraged in the future to detect medical conditions. For example, the results of the study will be useful, as the healthy breath VOC data from the sensor array can be cross-referenced in future studies aiming to use the device to distinguish disease states. Ultimately, the sensors may be integrated into a portable breathalyzer promoting rapid and noninvasive detection of medical conditions at home or in a point of care setting where access to traditional healthcare resources can be limited.
Conflict of Interest
Mangilal Agarwal has an ongoing collaboration with the NANOZ company and Scosche Industries to commercialize the sensors presented in this work for breath analysis and the detection of medical conditions. All other authors report no conflicts of interest relevant to this article.
Ethics Board Approval
All subjects provided written consent to participate in this study, and Institutional Review Board (IRB)/Ethics Committee approval was obtained (IRB # 12954). Institutional Biosafety Committee (protocol #IN-1301) approval was also obtained at Indiana University (IU).
|
Making an IMPACT: The results look promising for a P. aeruginosa breath test
Trenton J. Davis1,2, Brandie D. Wagner3, Jerry A. Nick4, Gary L. McPhail5, Jonathan H. Rayment6, Bradley S. Quon7, Sophia N. Williams8, Edith T. Zemanick9, Heather D. Bean1,2, and Jane E. Hill10
1School of Life Sciences, Arizona State University, Tempe, AZ, USA
2Center for Fundamental and Applied Microbiomics, Biodesign Institute, Tempe, AZ, USA
3Dept. of Biostatistics and Informatics, University of Colorado Anschutz Medical Campus and Children’s Hospital Colorado, Aurora, CO, USA
4Dept. of Medicine, National Jewish Health, Denver, CO, USA
5Dept. of Pediatrics, Cincinnati Children’s Hospital, Cincinnati, OH, USA
6Dept. of Pediatrics, BC Children’s Hospital, Vancouver, BC, CAN
7Dept. of Medicine, University of British Columbia, Vancouver, BC, CAN
8Dept. of Pediatrics, Phoenix Children’s Hospital, Phoenix, AZ, USA
9Dept. of Pediatrics, University of Colorado Anschutz Medical Campus and Children’s Hospital Colorado, Aurora, CO, USA
10Dept. of Chemical and Biological Engineering, University of British Columbia, Vancouver, CAN
Introduction
Chronic Pseudomonas aeruginosa (Pa) infections are associated with progressive lung function decline and increased risk of mortality in persons with CF (PwCF) [1]. Early detection of Pa is paramount for successful treatment [2], but we are arriving at a diagnostic cliff as we lose access to sputum due to the success of highly effective modulator therapies. Alternative airway samples (e.g., oropharyngeal swabs) have poor sensitivity for Pa lung infections [3]. We previously reported preliminary results from a clinical study titled “IMproving P. Aeruginosa deteCTion with Breath-based diagnostics” (IMPACT-Breath; NCT04735952) in which we showed that breath biomarkers are sensitive and specific for Pa infections, including in the setting of Staphylococcus aureus (Sa) co-infections, which are relatively common in pwCF. In this study we verified the reproducibility of our biomarkers using breath samples collected from independent subjects and analyzed at an independent site.
Methods
Breath samples (n = 163) were collected from multiple sites in the US and Canada and duplicate samples were analyzed at two sites using comprehensive two-dimensional gas chromatography and time-of-flight mass spectrometry. Sputum was also collected concurrently and cultured for typical CF lung pathogens, including P. aeruginosa and other common pathogens. Breath biomarkers were identified using machine learning tools, such as random forest (RF) and PLS-DA as well as statistical analyses.
Results
Approach 1. Unique patient samples at Site 1 were used to develop a model and different patient samples at Site 2 as the test set. For samples analyzed at Site 1, sputum culture detected Pa (n=15), Sa (n=30), and Pa/Sa co-infection (n=10); 27 samples had neither. Site 2 verification samples consisted of Pa (n=11), Sa (n=27), and Pa/Sa co-infection (n=6); 40 samples had neither. RF models classified Site 1 breath samples as Pa+/– with ≥98% sensitivity/specificity using as few as 10 VOCs. The inclusion of Sa co-infected patients lowered Pa classification accuracy to 91% sensitivity/specificity. Using the 10 most discriminatory VOCs for each model to classify the Site 2 samples resulted in ≥89% sensitivity and 100% specificity for Pa samples, with and without Sa co-infection. Approach 2. This time, site 2 samples (as above) were used to develop the model, which was then tested at Site 1 (using the unique samples, as above). The results from this approach also showed an accuracy of greater than 95%. Approach 3. Samples from all patients were combined and mined for breath biomarkers and the model was tested using leave one out cross-validation. The results from this approach also showed an accuracy of greater than 95%.
Table
From Approach 1: sensitivity/specificity of Pa breath biomarkers from RF models. Models were balanced by up-sampling the minority classes.
|
Sens. (%)
|
Spec. (%)
|
Site 1
|
|
|
Pa mono-infections
|
98.5
|
100.0
|
Pa w/Sa co-infections
|
91.2
|
91.2
|
|
|
|
Site 2 (verification)
|
|
|
Pa mono-infections
|
89.0
|
100.0
|
Pa w/Sa co-infections
|
90.5
|
100.0
|
Scroll/swipe this table left and right to see more information.
Conclusions
These results verify that a small subset of breath biomarkers are highly predictive for detecting Pa infection in the complex, polymicrobial environment of the CF lung.
References
[1] Gibson RL, Burns JL, Ramsey BW. Pathophysiology and management of pulmonary infections in cystic fibrosis. Am J Respir Crit Care Med. 2003;168:918
[2] Ratjen F, Moeller A, McKinney ML, Asherova I, Alon N, Maykut R, Angyalosi G, Early Study Group. Eradication of early P. aeruginosa infection in children <7years of age with cystic fibrosis: The early study. J Cyst Fibros. 2019;18:78
[3] Seidler D, Griffin M, Nymon A, Koeppen K, Ashare A. Throat swabs and sputum culture as predictors of P. aeruginosa or S. aureus lung colonization in adult cystic fibrosis patients. PLoS One. 2016;11:e0164232
Funding
This work was supported by the Cystic Fibrosis Foundation [Hill17P0, Hill18A0-CI] and the National Institutes of Health [R56HL139846]
|
TD-GC-EI-PTR-MS for Exhaled Breath Analysis
Anne E. Jung1, Alena R. Veigl1, Rhonda L. Pitsch2, Sean W. Harshman2
1 UES/Eqlipse Technologies, 711th Human Performance Wing, Air Force Research Lab, 2510 Fifth Street, Building 840, Area B, Wright-Patterson AFB, OH, USA
2 711th Human Performance Wing, Air Force Research Lab, 2510 Fifth Street, Building 840, Area B, Wright-Patterson FB, OH, USA
Introduction
In recent years, many advances have been made in the discovery and analysis of trace amounts of volatile organic compounds within exhaled breath for the non-invasive detection of disease and in physiological processes. New techniques and instrumentation that facilitate increased sensitivity and improved accuracy of unknowns have become of utmost importance. It is established that online exhaled breath analysis, via Proton Transfer Reaction–Mass Spectrometry (PTR-MS), illustrates high sensitivity coupled with accurate mass for the determination volatile compounds from a single exhalation. However, it is not always possible to field PTR instrumentation to remote locations. To allow for off-line remote sampling with PTR detection, individuals have coupled thermal desorption (TD) to PTR instrumentation (1). Here, we establish the use of duel MS data collection, both single quadrupole electron impact (EI) and PTR-MS detection, for the improved detection of volatile organic compound within exhaled breath.
Methods
A Markes International TD100xr was connected to two identical Restek RXI-624Sil MS columns (60m x 0.32mm, 1.8 mm (df)) with a glass Y-connector within a Thermo Scientific Trace Ultra GC. One column led to an ISQ single quadrupole mass spectrometer and the other exited the oven to a PTR-4000 (IoniON) mass spectrometer affixed with a nitrogen (99.999%) bag to compensate for the PTR flow. All desorbed volatiles were separated with a constant flow of 2.0 mL min-1 of helium into both mass detectors operated 30-350 amu (ISQ) and 5-390 amu (PTR). Gas standard mixtures containing 80+ compounds were purchased from Airgas and all TD tubes were Markes Biomonitoring (5TD) tubes. Data analysis was performed using a combination of the Thermo Xcalibur and the PTRMS Viewer software in addition to custom python scripts.
Results
Several aspects of the system were evaluated. First, the data indicate through analyses of the GC, TD cold trap, and adsorbent free TD tubes, that background contamination of acetaldehyde, acetone, methanol, and acetic acid were derived from the TD tube casings within both the EI and PTR data. Gas standards were utilized to identify a mean retention time shifts of 2.33±0.07min between detectors. The detection limits of both mass spectrometers, determined from gas standard mixes, indicate the EI instrument approaches low ppb levels (<1ppb) for select compounds while the PTR is less than 100ppt for several volatiles evaluated. Finally, preliminary exhaled breath data acquired from an ongoing exercise experiment within the lab suggests significant differences can be detected among the samples taken throughout an exercise regimen.
Discussion
Here, the use of TD-GC-EI-PTR-MS is established. We hypothesize with further research the dual acquisition will become standard for improved robust high sensitivity detection of exhaled breath volatiles.
The authors have no conflicts of interest to declare.
The views, opinions, and/or findings contained in this presentation are those of the author and should not be interpreted as representing official views or policies, either expressed or implied, of the Air Force Research Laboratory or the United States Department of Defense.
References
1. Romano A, Doran S, Belluomo I, Hanna GB. High-Throughput Breath Volatile Organic Compound Analysis Using Thermal Desorption Proton Transfer Reaction Time-of-Flight Mass Spectrometry. Anal Chem. 2018 Sep 4;90(17):10204-10210.
|
Addressing Complexity: The Evolution of Breath Biopsy and its potential in metabolic disease
Matt Kerr
Owlstone Medical, Ltd.
Within breathomics research, the importance of standardized and controlled breath sample collection is well-established. This talk will delve into the rationale behind recent advancements and modifications in Owlstone's breath sampling methodologies. We will then explore these advancements through the identification of potential volatile organic compound (VOC) biomarkers in type 2 diabetes. By integrating insights from the broader literature with our findings, we will examine how variations in collection and analytical techniques, as well as patient disease severity, may influence findings.
|
Micro-thermal Desorption Coupled to Gas Chromatography-Ion Mobility Spectrometry: Peppermint Protocol Standardization and Benchmarking
Kristian J. Kiland, Dorota M. Ruszkiewicz, Yoonseo Mok, Crista Bartolomeu, Scott A. Borden, Stephen Lam, and Renelle Myers
Integrative Oncology, BC Cancer Research Institute, Vancouver, Canada
Background
The Peppermint Initiative, established within the International Association of Breath Research, introduced the Peppermint Protocol, a breath analysis benchmarking initiative that seeks to address the lack of inter-comparability of outcomes across independent breath studies by standardizing approaches to breath sampling techniques and analytic platforms. The protocol includes a series of exhaled breath collections at defined sampling intervals up to 6 h after ingestion of encapsulated peppermint. The washout profiles of volatile terpenes from the peppermint oil are measured. The time to baseline is used as a benchmarking value and marker of the method’s sensitivity. Logarithmic peppermint washout curves allow for evaluation of the reproducibility of the method. Benchmarking of gas chromatography-mass spectrometry (GC-MS) and GC-ion mobility spectrometry (GC-IMS) using peppermint has been reported (Wilkinson et al. 2021, Ruszkiewicz et al. 2022). Coupling micro-thermal desorption (µTD) to GC-IMS has not been previously benchmarked in breath analysis.
Objective
To benchmark µTD-GC-IMS for breath analysis using the Peppermint Protocol.
Methods
Ten healthy participants (4 male and 6 female, aged 20 – 73 years), were enrolled to give six breath samples into Nalophan bags. Participants fasted prior to the baseline breath sample which was collected before the ingestion of encapsulated peppermint (Pepogest, Nature’s Way – 180 mg of pure peppermint oil). Breath sampling after peppermint ingestion occurred over 6 h at t = 60, 120, 200, 280, and 360 minutes. Participants were allowed to eat after the 200 min sample. After each breath collection, the Nalophan bag was immediately attached to the µTD-GC-IMS inlet for analysis. 120 cm3 of the breath sample was pre-concentrated in the µTD before being transferred into the GC-IMS for detection. One room air sample per participant was analyzed using the µTD-GC-IMS. To confirm the identities of peppermint compounds, permeation source standards of α-pinene, β-pinene, and eucalyptol were prepared and analyzed. Data was processed using VOCal, including background subtractions, peak volume measurements, and room air assessment.
Results
Four peppermint oil components were identified in post-capsule-ingestion breath samples: α-pinene, β-pinene, limonene, and eucalyptol. During peppermint washout, eucalyptol showed the highest change in concentration levels, followed by α-pinene and β-pinene. The reproducibility of the technique for breath analysis was demonstrated by constructing logarithmic washout curves, which were highly linear (average R2 = 0.93). The average time for a complete washout of eucalyptol (benchmark value for the µTD-GC-IMS) was 1111 minutes (95% CI: 529–1693 minutes), obtained by extrapolating the average logarithmic washout curve. This benchmark value is comparable to GC-MS, which had benchmark values ranging from 620–3300 minutes for eucalyptol washout. µTD-GC-IMS had a significantly higher (p-value = 4.577 x 10–5) benchmark value than the GC-IMS (367–484 minutes).
Conclusions
Using the Peppermint Protocol, we demonstrated that µTD-GC-IMS is reproducible and suitable for breath analysis. We obtained a benchmark value (eucalyptol washout) for the µTD-GC-IMS of 1111 minutes (95% CI: 529–1693 minutes) for eucalyptol, which is comparable to the gold standard GC-MS. Notably, it was more than double the benchmark value for GC-IMS, indicating increased sensitivity with this method.
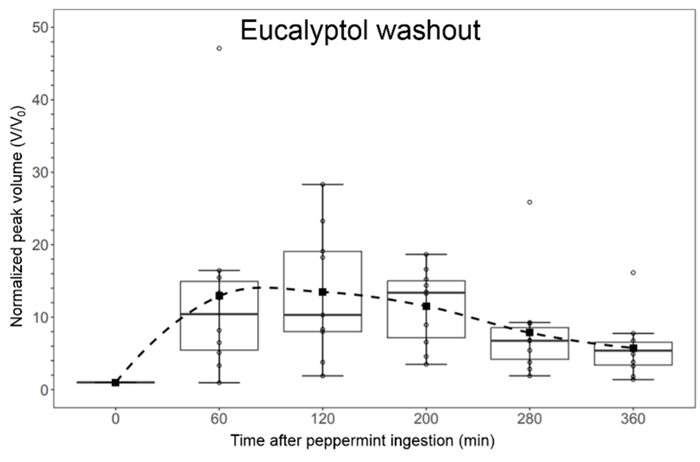
Figure 1. Eucalyptol washout measured via the micro-thermal desorber-gas chromatograph-ion mobility spectrometer (µTD-GC-IMS).
|
Human breath volatilome: a comparison of sampling and mass spectrometry methods for biomarker discovery in clinical research
Elodie Lamy1, Camille Roquencourt2, Nicolas Hunzinger1, Philippe Devillier1, 2, Emmanuelle Bardin1, 2, 3, Julie Mercier1, Stanislas Grassin-Delyle1, 2
1Université Paris-Saclay, UVSQ, INSERM, Infection et inflammation (2I), U1173, Département de Biotechnologie de la Santé, Montigny le Bretonneux, France
2Hôpital Foch, Exhalomics®, Suresnes, France
3Institut Necker-Enfants Malades, Paris, France
Background
Precision medicine lacks non-invasive biomarkers that can be measured using high-performance, rapid techniques. Volatilomics, an 'omics' approach, focuses on analyzing breath volatile organic compounds (VOCs). Mass spectrometry (MS) serves as the reference technology, but various types of mass spectrometers can be employed, leading to the generation of signals with diverse characteristics and levels of exhaustiveness. The aim of this study was to compare the volatilomic information obtained from online or offline mass spectrometry techniques in clinical studies.
Methods
We conducted a single-center clinical study in a university hospital (VOC-Compare, NCT06020521). Breath samples from 40 healthy participants were analyzed with three real-time MS instruments: PTR-Qi-TOF and PTR-TOF 10k (proton transfer reaction – mass spectrometry, Ionicon), as well as with a Soft Ionization by Chemical Reaction in Transfer (SICRIT®, Plasmion) ion source coupled to a Q-exactive MS (Thermofisher) (3 exhalations each). Breath was also collected on sorbent tubes (Tenax TA, Markes) using both a ReCIVA device (Owlstone) and sampling bags (Tedlar® bag, SKC) (0.5 L/tube) for subsequent thermal desorption and bidimensional gas chromatography – mass spectrometry (TD-GCxGC-MS) analysis (BT4D, Leco).
Results
A total of 120 breath samples were obtained. Using real-time analysis, 104 features were detected with the PTR-Qi-TOF (mass range 28-371 m/z), 252 with the PTR-TOF 10k (28-373 m/z), and 586 with the SICRIT-HRMS (51-294 m/z) after data filtering. High reproducibility was achieved reaching 7%, 6%, and 19% intra-participant variability, respectively.
GCxGC-MS provided 999 and 1144 filtered features (40-596 m/z) in samples collected with Tedlar® bags and ReCIVA, respectively. Annotated features accounted for 86% and 89% of detected features, respectively, with 34% features in common.
Conclusions
Each MS technique comes with its own set of advantages and limitations in terms of implementation and performance. The nature and completeness of the information obtained varies among them, suggesting potential benefits in selecting a technique based on its unique advantages or in combining several of them to enhance the completeness of the information generated during clinical studies aimed at discovering biomarkers.
Fundings
This work was supported by Région Île de France (VolatolHom, SESAME 2016 and MeLoMane, DIM 1HEALTH 2019), Saint-Quentin en Yvelines (ESR 2021) and Fondation Foch (VolatolHom and VOC-Info).
|
A state-of-the-art laser technology for detecting breath molecules
Qizhong Liang and Jun Ye
JILA
A laser technology built upon Nobel-winning technology (2005, Physics) has recently been tested its utility for breath-based diagnostics for the first time [Liang et al 2023 JBR; Liang et al 2021 PNAS]. In a trial study over 170 research subjects, breath tests using this laser technology reported a matching rate of 85% to the standard RT-PCR tests. In this talk, we will give a general introduction to its working principle, showing how we can detect multiple molecular species in a breath sample free from chemical reactions and down to parts-per-trillion detection sensitivity.
|
Defining VOC Signatures of Airway Epithelial Cells After Environmental Exposures
Angela M. Linderholm1,2, Kat Aribindi1, Eva Borras3, Katie Hamera3, Keith Bein5, Mitchell M. McCartney3,4, Cristina Davis3,4, Richart W. Harper1,2,4, Nicholas J. Kenyon1,2,4
1Division of Pulmonary, Critical Care and Sleep Medicine, University of California, Davis, Sacramento, CA
2Lung Center, University of California, Davis, Davis, CA
3Mechanical and Aerospace Engineering, University of California, Davis, Davis, CA
4VA Northern California Health Care System, Mather, CA
5Air Quality Research Center, University of California, Davis, Davis, CA
Background
We proposed to identify volatile organic compounds (VOCs) produced by the lung upon exposure to environmental pollutants to develop an exhaled breath signature that will be utilized for future clinical studies. Previously, we developed a reliable method to measure VOCs emitted from well-differentiated tracheobronchial epithelial cells in vitro. Using this method, we exposed well-differentiated small airway and bronchial airway epithelial cells to varying doses of traffic-related air pollutants (TRAP) to determine specific VOC signatures after exposure to TRAP. We utilized TRAP collected from the Caldecott tunnel in Oakland, CA to model “real-life” exposures.
Methods
Human bronchial/tracheal airway epithelial (BAE) cells and small airway epithelial (SAE) cells were obtained from Lifeline Cell Technology (Frederick, MD). The BAE and SAE cells were plated on Transwell (Corning Costar, Corning, NY) chambers (12 mm) at 1–2 × 104 cells/cm2 coated with 0.05mg/mL type IV collagen (Sigma) in the PneumaCult-Ex medium (Stemcell Technologies). Once BAE and SAE cultures were confluent, they were transferred to ALI culture conditions in their respective media (Stemcell Technologies) for 1 month. Transwells containing confluent cells were placed into glass jars filled with 5mL of the appropriate media and capped with lids that had Twisters magnetized to them. The VOCs were extracted from the Twisters and analyzed using mass spectrometry. We also collected media samples to measure IL-6 and IL-8 protein levels secretion by ELISA.
Results
Exposure to TRAP resulted in distinct VOC and IL6 and IL8 responses, that differed between BAE and SAE cells, as well as individual subjects with little overlap. The VOCs produced by cells included aldehydes, ketones and hydrocarbons that previously have been linked to oxidative stress pathways.
Conclusions
Our studies suggest that TRAP exposure induced a specific cellular response, unique to BAE and SAE cells, related to their different functions that can be exploited for future clinical studies. We will further explore this phenomenon in future in vitro studies and pursue identification of the VOCs to assess metabolic pathways of importance.
|
Chip-Scale Mass Spectrometry for Point-of-Care Breath Diagnostics
Spiros Manolakos, Ashish Chaudhary, Ph.D., Kelli Barr, Ph.D., Christina Davis, Ph.D.
Detect-ION, University of South Florida, University of California - Davis
Background
The recent COVID-19 pandemic has underscored the urgency of swiftly identifying infected individuals to intervene early and prevent the progression to severe illness, as well as to curb the transmission of infections within communities. Exhaled breath diagnostics can provide such rapid screening approach while being non-invasive, cost-efficient, and potentially adaptable to detect various infectious agents, sometimes even before symptoms appear. Traditional breath analysis methods often entail collecting breath samples using tubes or bags for transportation to labs where Gas Chromatography-Mass Spectrometry (GC-MS) is employed for detecting trace-level organic compounds. While considered the gold standard, this method is both costly and logistically cumbersome. To address this, Detect-ION has leveraged its cutting-edge "chip scale mass spectrometry" technology, enabling a compact 10-L Preconcentrator-Thermal Desorption-Gas Chromatograph-Mass Spectrometer (TD-GC-MS) system, called “CLARION”, for analyzing exhaled breath.
Objective
In CLARION, our goal is to identify the relevant VOC biomarkers in exhaled breath that distinguish between infected individuals and a healthy population. If successful, a single CLARION device could require no more than a 1-minute breath sample per individual and conduct up to 160 breath analyses per day. Moreover, this approach could be more cost-effective than rapid PCR tests, enabling early detection of infections and facilitating high-throughput screening in large populations.
Methods
In CLARION project, we have established an institution review board (IRB) and plan to enroll asymptomatic and symptomatic human subjects across two sequential breath collection campaigns of cohort sizes of 100 (Campaign-1) and 500 (Campaign-2) respectively. Human subjects enrolled for the study will provide breath specimens, as well as nasal and throat swabs. Rapid antigen tests will evaluate the specimens for influenza, SARS-CoV-2, RSV, and group A streptococcus. In addition, RT-PCR will also be performed as a secondary diagnostic to validate antigen testing, with plans to perform BioFire® Respiratory 2.1 panel to accurately detect and identify the pathogens most associated with respiratory infections. Breath samples will be collected into the portable CLARION device for analysis, and into Tedlar bags and sorbent tubes for laboratory analysis on a commercial benchtop GC-MS. GC-MS data from the CLARION platform will be processed to generate a fingerprint pattern consisting of calibrated retention times and chemical identities for each VOC peak. These patterns along with the controls, which categorize each subject as uninfected or infected, will be provided as input to a Partial Least-Squares Discriminant Analysis (PLSDA) model building tool to develop detection algorithms.
Preliminary Results
The IRB has been accepted and we plan to begin enrollment for Campaign-1 in June 2024. A miniature breath collector with an embedded thermal desorption stage has been developed and
successfully integrated into the CLARION platform. The MBC has smart collection technology to perform volumetric sampling of breath. Initial studies of vapor phase analytical standards have shown sensitivity of the system in the low parts-per-billion to high parts-per-trillion range.
Conclusion
Fieldable GC-MS system adapted to collect exhaled human breath can provide a versatile diagnostics capability for rapid screening of a large population.
|
From research to routine: Meeting the evolving demands of clinical breath analysis with advancements in thermal desorption
Helen Martin1, Laura Miles1, Ryan Francis1, James Swift2 and Matthew Turner2
1Markes International Ltd, 1000B Central Park, Western Avenue, Bridgend, CF31 3RT, UK
2Department of Chemistry, Loughborough University, Loughborough, LE11 3TU, UK
Breath-based biomarker discovery research is entering a particularly exciting phase with many studies scaling up to large clinical trials and generating viable candidate marker compounds. As the size of sample sets increases, so too does the duration of individual studies and the number of personnel involved. This scale-up of efforts introduces more opportunities for error and highlights the need for rigorous quality control.
Thermal desorption (TD) coupled with gas-chromatography mass-spectrometry (GC-MS) has long been established as the gold standard technique for breath analysis. Volatile organic compounds (VOCs) in breath are pre-concentrated by collection onto sorbent packed tubes and shipped to a central laboratory for analysis. These tubes are small, physically robust, easy to transport and provide extended sample storage stability compared to other methodologies.
In this presentation we will discuss how quality control strategies can be built into thermal desorption-based workflows throughout the life cycle of every sample tube. These strategies include the use of leak testing, surrogates and internal standards and automated water management. We will explore the benefits of re-collecting a portion of breath samples for further analyses and show how this re-collection can reduce the sampling burden, aid sample archiving and extend the dynamic range of the analysis to help manage the wide-ranging concentration of important breath components.
We will also review the generation of pooled biological quality control samples using re-collection and how they could be applied to proficiency testing and longitudinal studies. Pooled quality control samples are a core concept in metabolomic studies, providing a representative sample matrix and metabolite composition. However, these very useful and informative samples are not easily produced or integrated into breath VOC workflows. To demonstrate the concept of a pooled quality control sample for breath, we utilise the protocol described in the peppermint experiment (Henderson et al 2020). Here, multiple breath samples are collected over a 10-hour period after the ingestion of a peppermint oil capsule. The resulting breath samples contain several VOCs including menthol, menthone and alpha-pinene which exhibit a range of concentrations in different participants at the various time points.
We will explore how these pooled breath samples can be applied to routinely evaluate data quality, reproducibility of the method and instrumentation, and correct for any system bias over time.
1. Ben Henderson et al 2020 J. Breath Res. 14 046008
|
Selectivity to diagnose COVID-19 infection from exhaled breath volatiles (EBV) against other common upper respiratory infections
Mitchell M McCartney, Eva Borras, Dante E Rojas, Tiffany Lam, Enrique Lopez, Nicholas J Kenyon, Cristina E Davis
Mechanical and Aerospace Engineering; UC Davis Lung Center; and the Department of Internal Medicine, UC Davis, Davis, California. VA Northern California Healthcare System, Mather, California.
Background
Many studies have reported that COVID-19 infection can be identified through metabolites in exhaled breath vapor (EBV). However, during the first two years of the COVID pandemic, there was a stark decline in incidences of other respiratory infections, such as influenza and RSV, which nearly disappeared globally. Without transmission of non-COVID pathogens, it was not possible to compare the selectivity of a breath-based test to identify COVID+ patients against other common upper respiratory infections. Thus, it was unknown if the reported shift in EBV from COVID-19 was a unique signature to COVID, or whether COVID breath tests might be confounded by a general response to airway infection as observed in breath.
Objective/Aims
The objective of this study was to collect EBV samples from patients with known respiratory infections, including coronaviruses, influenzae, and RSV, to determine the selectivity of a breath-based test to diagnose COVID-19.
Methods
Breath vapor samples were collected from patients exhibiting symptoms of an upper respiratory infection. A nasal swab was also collected and analyzed using the BioFire Respiratory 2.1 Panel, which targets 22 viruses and bacteria, including SARS-CoV-2. Those positive for SARS-CoV-2 were phenotyped, as we previously reported that major shifts in COVID variant (eg, from Delta to Omicron) significantly altered the COVID breath signature.
Collection occurred at the UC Davis Medical Center and from the surrounding Sacramento community under an IRB-approved protocol, #1636182. Demographic information about volunteers was collected through a questionnaire and review of electronic medical records.
Breath was collected in Tedlar bags, then extracted onto Tenax TA sorbent tubes. A paired background/environmental air sample was collected for each breath sample to remove exogenous features from the corresponding breath sample.
Samples underwent thermal desorption-gas chromatography-mass spectrometry (TD-GC-MS) analysis. GC-MS data were deconvoluted and aligned. Background VOCs were subtracted from breath samples. The dataset was randomly split into a training and validation set for machine learning algorithms to identify the COVID-19 breath signature.
Results / Conclusion
At time of abstract submission, sample collection and chemical analysis are ongoing, with 177 negative controls enrolled, 147 COVID+, 4 influenza, 2 rhinovirus, and 47 samples from symptomatic patients pending analysis of their nasal swab. During the IABR Meeting, the authors will present the latest findings, detailing the accuracy, sensitivity and specificity of a breath-based GC-MS assay to diagnose COVID-19 against other respiratory pathogens. We will present findings on whether COVID-19 biomarkers correlated with demographic factors such as age, ethnicity, or correlated with disease severeness and symptoms.
|
Peptides and nanotubes - a machine learning and biological approach to VOC sensing
Oliver Nakano-Baker, Richard V. Lee, Shalabh Shukla, J. Devin MacKenzie
University of Washington Materials Science & Engineering, University of Washington Laboratory Medicine & Pathology, University of Washington Mechanical Engineering
Background
Volatile organic compounds (VOCs) in breath are promising clinical biomarkers for various diseases, but detecting VOCs outside of the lab presents challenges. Portable eNoses struggle to match the sensitivity and specificity of mass spectroscopy to key VOC markers of disease. We recently demonstrated an alternate approach to portable breath fingerprinting: a bioelectronic platform that combines carbon nanotube (CNT) sensors with surface-binding peptide molecules derived from olfactory proteins.
Objective: Here, we propose a pipeline to design, combine, and fabricate micro-scale biomimetic sensors for specific VOCs. These sensors are the building blocks of eNoses that are capable of performing targeted breath-based diagnostics. Ideally, the pipeline translates a VOC fingerprint of a disease to a fabricated multiplex chip capable of detecting the fingerprint. It is demonstrated here by creating a sensor for ethyl butyrate, a VOC marker of bacterial infections and COVID-19 disease.
Methods: Sensor design proceeds in three steps. First, bioinformatics and molecular dynamics identify peptide probes with binding affinity to certain VOCs, creating a library of targeted binders. Second, bootstrapped machine learning models evolve the peptide composition of a multi-channel eNose to maximize the ability to classify disease fingerprints. Finally, CNT field-effect transistors are incubated with the engineered peptides to sense VOCs by direct signal transduction with sub-parts-per-billion sensitivity.
Results
A multiplex sensor consisting of just 5 peptide-CNT transistors is capable of identifying the VOC fingerprint of COVID-19 in the bootstrapped design loop, and a 10-plex design extends this capability to multiple respiratory diseases, including influenza, rhinovirus, and asthma. All engineered probes display selective binding to their VOC targets as measured by quartz crystal microbalance, and a CNT transistor utilizing a peptide probe that targets the VOC ethyl butyrate exhibits p<0.001 stronger source-drain current when its target is present, compared to a control at the same concentration.
Conclusion
With promising analytical performance and an expansive probe design space, the biomimetic eNose promises a step-change in the sensitivity and specificity of micro-scale VOC detection. If challenges of durability and human variability can be addressed, it could be the key to mobile breathomics - phone-size breath sensors capable of multi-viral diagnoses, blood glucose monitoring, cancer screening, and more.
Disclosures
Research was performed at the University of Washington (UW) under the WE-REACH program through the RADx RAD program at NIDCR/NIH and the Washington Research Foundation. Additional funds were provided by UW CoMotion, the School of Dentistry, and NSF grant 2313269. Authors Nakano-Baker, Lee, and Shukla are co-founders of Odo Labs, LLC.
|
From dynamic nature to human origin of breath isoprene – an investigative story
Pritam Sukul1, Anna Richter2, Christian Junghanss2, Jochen K Schubert1, Wolfram Miekisch1
1Rostock Medical Breath Research Analytics and Technologies (ROMBAT), Dept. of Anesthesiology, Intensive Care Medicine and Pain Therapy, University Medicine Rostock, Rostock, Germany,
2Department of Medicine, Clinic III – Hematology, Oncology, Palliative Medicine, Rostock University Medical Center, Rostock, Germany
Background
Isoprene (1,3-pentadiene, C5H8) is amongst the most abundant volatile metabolites produced by plants and animals. In humans, endogenously produced isoprene is exhaled in nearly everybody’s breath in high amounts and has been described as a potential biomarker for various diseases1. Exhaled isoprene is extremely dynamic in nature2 and concentration changes are also related to physio-metabolic, inherited conditions and ageing3–5. Based on pre-clinical in vitro experiments, Deneris et al hypothesized that cholesterol biosynthesis in the liver potentially gives rise to isoprene in our breath6. Nevertheless, changes and/or differences observed in breath isoprene under various conditions investigated in clinical studies could not be justified by the suggested origin. Thus, missing knowledge of the origin and pathways involved hindered the translation of this biomarker into clinical practice. Isoprene absent healthy adults is rare (<0.3/%) in the nature. Our previous investigations in such a healthy German adult and her family (isoprene deficient) disqualified the putative origin and depicted a recessive inheritance of this phenotype7.
Objective
We aimed to find the human metabolic origin of isoprene8.
Methods
In consecutive clinical breath screening studies, amongst 2000 subjects, we identified five healthy German adults without breath isoprene. After having the ethical clearance, we conducted venous blood collection, peripheral blood mononuclear cells isolation, DNA isolation, multi-omics (breathomics, genomics) and serological metabolites analysis in healthy isoprene aberrated and isoprene normal adults8.
Results
We obtained five (3 females and 2 males) isoprene absent healthy adults, 64 (39 females and 25 males) isoprene deficient (with low exhaled concentrations <50ppbV). Whole exome sequencing in these individuals revealed only one shared homozygous (<1% prevalent in Europeans) IDI2 stopgain mutation (at c.431 position) causing loss of enzyme active site and Mg–cofactor binding sites and thereby, preventing conversion of isopentenyl diphosphate to dimethylallyl diphosphate (DMAPP) in cholesterol metabolism. Targeted sequencing depicted that the IDI2 variant is heterozygous in isoprene deficient blood-relatives and absent in unrelated isoprene normal adults. Wildtype IDI1 and cholesterol metabolism related serological parameters were normal in everyone. Unlike other mammals, naturally IDI2 knocked out pigs and bottlenose dolphins exhale no isoprene. Human IDI1 is expressed highly in liver but the hepatocellular cytochrome P450 enzymes immediately oxidizes isoprene. Skeletal muscles metabolize lipids (oxidize cholesterol, fatty acids) to produce energy, regulate intramyocellular signalling and integrity. Peroxisomal beta-oxidation produces acetyl-CoA i.e., channelled towards DMAPP production. Thus, human IDI2 determines isoprene production as DMAPP is the only source of isoprene and unlike plants, humans lack isoprene synthase and its enzyme homologue. Human IDI2 is only expressed in skeletal-myocyte peroxisomes and instant spikes in isoprene exhalation during any muscle activity underpin the origin from muscular lipolytic cholesterol metabolism.
Conclusions
Our discovery of the genetic origin and metabolic routes of human isoprene production enabled objective interpretations and applications of isoprene as a noninvasive biomarker. Knowledge of accurate metabolic source and normal/dynamic range are indispensable for valid clinical interpretation of any endogenous marker. Besides isoprene, our breath contains many potentially endogenous VOCs and their origins are yet uncertain.
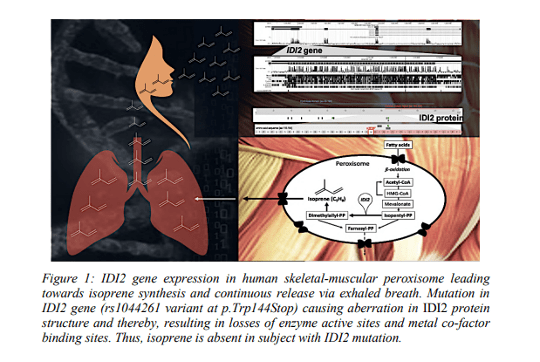
References
1. Mochalski, P., King, J., Mayhew, C. A. & Unterkofler, K. A review on isoprene in human breath. J. Breath Res. (2023) doi:10.1088/1752-7163/acc964.
2. Miekisch, W., Sukul, P. & Schubert, J. K. Chapter 2 Origin and Emission of Volatile Biomarkers in Breath: Basics and Dynamic Aspects. in Volatile Biomarkers for Human Health: From Nature to Artificial Senses 22–38 (The Royal Society of Chemistry, 2023). doi:10.1039/9781839166990-00022.
3. Sukul, P. & Trefz, P. Physio-Metabolic Monitoring via Breath Employing Real-Time Mass Spectrometry: Importance, Challenges, Potentials, and Pitfalls. in vol. 4 1–18 (Springer, 2022).
4. Hoffmann, G. et al. Mevalonic Aciduria — An Inborn Error of Cholesterol and Nonsterol Isoprene Biosynthesis. New England Journal of Medicine 314, 1610–1614 (1986).
5. Sukul, P. et al. Physiological and metabolic effects of healthy female aging on exhaled breath biomarkers. iScience 25, 103739 (2022).
6. Deneris, E. S., Stein, R. A. & Mead, J. F. Invitro biosynthesis of isoprene from mevalonate utilizing a rat liver cytosolic fraction. Biochemical and Biophysical Research Communications 123, 691–696 (1984).
7. Sukul, P., Richter, A., Schubert, J. K. & Miekisch, W. Deficiency and absence of endogenous isoprene in adults, disqualified its putative origin. Heliyon 7, e05922 (2021).
8. Sukul, P., Richter, A., Junghanss, C., Schubert, J. K. & Miekisch, W. Origin of breath isoprene in humans is revealed via multi-omic investigations. Commun Biol 6, 1–12 (2023).
|
Developing novel perovskite-based nanomaterials for the detection of volatile biomarkers in exhaled breath
Y.L. Pham1, J. Beauchamp1, A. Kostopoulou2, K. Brintakis2, E. Stratakis2, L. Manna3
1Fraunhofer Institute for Process Engineering and Packaging IVV, Giggenhauser Str. 35, 85354 Freising, Germany.
2Foundation for Research and Technology – Hellas (FORTH), Ν. Plastira 100, Vassilika Vouton, GR - 700 13, Heraklion, Greece.
3Fondazione Istituto Italiano di Tecnologia (IIT), Via Morego, 30, 16163 Genoa, Italy.
Abstract
Comprehensive laboratory-based analytical devices pose limitations due to the skills and training required for operation, rendering it impractical for routine use in breath testing. Additionally, these devices are not suitable for therapeutic monitoring or wearable diagnostic applications. Therefore, there is a pressing need to miniaturize breath analysis sensors to achieve a low-cost solution with high sensitivity, selectivity, and rapid response time for detecting volatile organic compounds (VOCs) at low concentrations. However, the development of breath-sensing materials is a complex task that demands expertise in both materials development and breath analysis. This duality is essential for creating proof-of-concept sensors for detecting exhaled breath markers for targeted applications.
A current interdisciplinary European study aims to promote new opportunities for participating partners (FORTH, IIT, FHG) to improve the levels of excellence and expertise of all three institutions in the fields of novel perovskite nanocrystals for sensing applications and breath-based diagnostics. Over the course of the project, perovskite sensing elements are synthesized and characterized, which are imperative for the fabrication of breath sensing devices. Currently, different structures of perovskite-based nanocrystals are tested for the detection of selected VOCs. The sensing ability for breath VOCs will be then evaluated in simulated conditions using different target volatiles relevant to the peppermint experiment1, 2 – a benchmarking initiative established by the International Association of Breath Research (IABR) that seeks to address the lack of inter-comparability of outcomes across independent breath biomarker studies. Once established, this system will be implemented in the peppermint experiment with real breath samples to obtain benchmark values for the perovskite-based nanomaterials to establish this technology in breath research.
The poster outlines the project concept for the development and validation of novel breath-sensing devices, the materials and methods being employed, and intends to spark debate on novel perovskite-based nanomaterials for the detection of breath volatiles.
References
1Henderson, B. et al. A benchmarking protocol for breath analysis: the peppermint experiment. J. Breath Res.14.4 (2020): 046008.
2Pham, Y. L., Yu, R., Beauchamp, J. Cross-validation of the peppermint benchmarking experiment across three analytical platforms. J. Breath Res. 17 (2023): 046003.
|
Interest of Soft Ionization by Chemical Reaction in Transfer (SICRIT) – high resolution mass spectrometry for exhaled breath analysis in clinical studies
Camille Roquencourt1, Elodie Lamy2, Nicolas Hunzinger2, Hélène Salvator3, 4, Philippe Devillier1, 4, Emmanuelle Bardin1, 2, 5, Stanislas Grassin-Delyle1, 2
1Hôpital Foch, Exhalomics®, Suresnes, France
2Université Paris-Saclay, UVSQ, INSERM, Infection et inflammation (2I), U1173, Département de Biotechnologie de la Santé, Montigny le Bretonneux, France
3Service de pneumologie, Hôpital Foch, Suresnes
4Laboratoire de recherche en Pharmacologie Respiratoire – VIM Suresnes, UMR 0892, Université Paris-Saclay, Suresnes, France
5Institut Necker-Enfants Malades, Paris, France
Background
Real-time mass spectrometry (MS) breath analysis is a non-invasive, rapid method for disease diagnostic and treatment monitoring that relies on the detection of volatile organic compounds (VOC). A novel approach for real-time VOC analysis involves an ambient flow-through ionization technique, using Soft Ionization by Chemical Reaction in Transfer (SICRIT) - mass spectrometry with atmospheric pressure inlet.
Objective
The objective of the study was to assess the feasibility of implementing exhaled breath analysis by SICRIT-MS within the context of clinical studies.
Methods
We conducted a clinical study within a university hospital (VOC-Compare, NCT06020521). Main inclusion criteria were adult healthy volunteers and non-inclusion criteria were pregnancy, any chronic condition and smoking. Measurements included breath analysis with SICRIT-MS (Plasmion), among other techniques. SICRIT parameters were set at 1600 V and 15000 Hz. VOC detection was carried out using a Q-Exactive mass spectrometer (Thermofisher) in positive ionization mode, with full-scan data acquisition in the range 50-300 m/z. Sheath and auxiliary gas flow rates were set at 0 and 12 arbitrary units, respectively. The capillary temperature was maintained at 200°C, and the S-Lens RF level was set at 65. Participants performed three consecutive expirations through disposable mouthpieces into the device, aiming to sustain an expiration airflow at 8 L/min, which was simultaneously assessed in real-time. The primary outcome measure was the number of reliable individual signals detected. Data analysis was performed using an in-house R script, involving mass calibration, peak detection in the mass dimension at each time point, alignment of peaks in the time dimension, and the selection of compounds specifically detected in the expiration phases.
Results
Breath samples from 40 healthy participants were successfully analyzed. Preliminary data analysis was conducted, and expiration phases were accurately identified using isoprene as a tracer (m/z 69.07). Calculated resolution on the three calibration peaks (m/z 67.069, 149.044 223.063) was in the range of 75,000 to 130,000. The total average feature number per sample was 1090, with 59% of features significantly more abundant in expiration phases than in the background. After alignment and isotope suppression, a total of 586 features (mass range: m/z 51-294, mean signal to noise ratio: 45) were detected in more than 30% of participants' breath samples, including 417 features detected in the breath of all individuals.
Conclusion
Our results demonstrate the successful implementation of SICRIT-MS breath analysis in clinical studies, highlighting its potential as a means for real-time biomarker discovery in breath utilizing high-resolution mass spectrometry detection.
|
Investigating Exhaled Volatile Organic Compounds in Healthy Breath Using Solid Phase Microextraction and Gas Chromatography-Mass Spectrometry
Eray Schulz1,2, Shivaum Heranjal3, Mariana Maciel1, Sha Cao4, Xiaowen Liu5, Mark Woollam1,2, and Mangilal Agarwal1,2,3,6
1Chemistry and Chemical Biology, Indiana University Indianapolis, IN, United States.
2Integrated Nanosystems Development Institute, Indiana University Indianapolis, IN, United States.
3Electrical and Computer Engineering, Purdue University Indianapolis, IN, United States.
4Biostatistics and Health Data Science, Indiana University School of Medicine, Indianapolis, IN, United States.
5Biomedical Informatics and Genomics, Tulane University School of Medicine, New Orleans, LA, United States.
6Biomedical Engineering and BioHealth Informatics, Luddy School of Informatics, Indiana University Indianapolis, IN, United States.
Background
Volatile organic compounds (VOCs) in exhaled breath are potential biomarkers that may facilitate non-invasive health monitoring in diverse locations including at-home. One challenge that still exists however, is that baseline levels of VOCs in relatively healthy breath have not been benchmarked.
Objective
This study aimed to identify baseline levels of VOCs in exhaled breath using solid phase microextraction (SPME) and gas chromatography – mass spectrometry (GC-MS).
Methods
An optimized and standardized direct breath sampling method using solid-phase microextraction (termed DB-SPME) coupled to gas chromatography – mass spectrometry (GC-MS) was implemented to analyze samples collected from 164 “healthy” volunteers. 10 of these volunteers provided an additional 9 samples longitudinally over the course of 6 months. Blood samples for comprehensive metabolic panels and complete blood counts were collected, as well as data from a food diary and lifestyle questionnaire to obtain information on confounding variables that may impact “healthy” VOC levels.
Results
After instrumental analysis, data processing procedures were implemented (removing SPME artifacts, subtracting background air, etc.) which identified a total of 62 VOCs including terpene/-oids, carbonyls, aromatics and other functional groups. Hierarchical clustering showed cyclic monoterpenes had similar trends in healthy breath samples with a significant correlation of specific monoterpenes including α-pinene and β-pinene (R2=0.58, p=1x10-29), pointing to a relationship between structure and expression. Reproducibility was also benchmarked, showing cross-sectional VOC measurements were significantly more variable compared to those collected longitudinally. Correlation analyses of VOCs and confounding variables showed male volunteers had significantly elevated levels of breath isoprene relative to women (p=0.04), corresponding to previous literature. Along with this, acetone and isoprene breath signals were quantified using calibration curves developed from a previous study. Acetone and isoprene concentrations ranged from 21ppb to 773 ppb and 58ppb to 1734ppb in the cross-sectional cohort respectively. Longitudinal concentration ranges were lower, with acetone being between 22ppb-343ppb, and isoprene between 38ppb-983ppb.
Conclusion
In the future, the results from this work can be leveraged to solidify our understanding of baseline levels of VOCs in breath that can be applied to disease-specific studies. Furthermore, continuing to quantify VOC signals can help push the field of breath biopsy to more clinically translatable applications.
Conflict of Interest
Mangilal Agarwal has an ongoing collaboration with the NANOZ company and Scosche Industries to commercialize sensors to detect VOCs presented in this work for noninvasive health monitoring applications. All other authors report no conflicts of interest relevant to this article.
Ethics Board Approval
All subjects provided written consent to participate in this study, and Institutional Review Board (IRB)/Ethics Committee approval was obtained (IRB # 12954). Institutional Biosafety Committee (protocol #IN-1301) approval was also obtained at Indiana University (IU).
|
Extending Laser Absorption Spectroscopy towards Detection of Larger Volatile Organic Compounds in Breath
Miloš Selaković1,2, Lukas Emmenegger1, Renato Zenobi2, Béla Tuzsona1
1Laboratory for Air Pollution/Environmental Technology, Empa, Überlandstrasse 129, 8600 Dübendorf, Switzerland
2Department of Chemistry and Applied Biosciences, ETH Zürich, Vladimir-Prelog-Weg 1−5/10, 8093 Zürich, Switzerland
Background
Laser absorption spectroscopy (LAS) is well-established for trace gas measurement of small, inorganic molecules, providing fast and accurate response in relatively compact, easy-to-use, and cost-effective instrumentation. Extending LAS towards the detection of volatile organic compounds (VOCs) would be highly beneficial for future point-of-care diagnostics based on human breath. However, larger VOCs often exhibit broad spectral absorption features which are usually attributed to the overlap of a multitude of absorption lines from many vibrational modes, and therefore limiting the LAS to detection of VOCs with molar mass <40 g/mol. [3] Despite this common view, we recently developed a compact mid-infrared (mid-IR) analyzer for simultaneous measurement of small VOCs in breath. [1,2] In this work, we show that even larger molecules (with a molar mass over 100 g/mol) can be measured by LAS.
Methods
We used a custom-built spectrometer based on a widely electrically tunable quantum cascade laser that allows access to six different spectral windows around 1080 cm–1 wavenumber. [1] VOC gas standards were generated in the laboratory by vaporization of liquid samples into a carrier gas stream in a dynamic injection design [4]. The samples were analyzed in a flowthrough configuration at low gas pressure (~50 mbar) to reduce the broadening of the absorption lines. The high-resolution spectra of pure substances were used for the generation of a reference database.
Results
By extensive validation of our method through the series of spiking experiments, we demonstrated that our instrument is well-suited for the quantification of oxygen-containing VOCs at amount fractions down to tens of ppb. The broad measuring range, high spectral resolution, and the unique spectral fingerprints of the investigated VOCs assure excellent selectivity of the method and enable multi-compound measurements in the breath. With a time resolution of 360 ms, concentration profiles of several VOCs, water and CO2 in one breath stroke can be retrieved with a relative expanded uncertainty (k=2) of <2%.
Spectral screening of over 50 different VOCs revealed significant fine structure not only in the ro-vibrational spectrum of small (up to 4 non-hydrogen atoms) but also of large molecules (6 or more non-hydrogen atoms) with a rigid and symmetrical chemical structure. To investigate the effect of molecular symmetry and rigidity on spectral fine structure in mid-IR, several exemplary compounds with similar molar mass but different structures have been investigated. One such example is shown in Figure 1. We argue that the absence of spectral fine structure in heavy linear-chain low-symmetry molecules is related to a large number of available con- formers where each conformer contributes to the complexity of the spectrum. We demonstrated that our method can capture different conformers of a compound in the example of Nmethylformamide. These findings have the potential to substantially increase the number of possible VOCs that can be analyzed with LAS and open up the path towards other applications, such as conformational analysis.
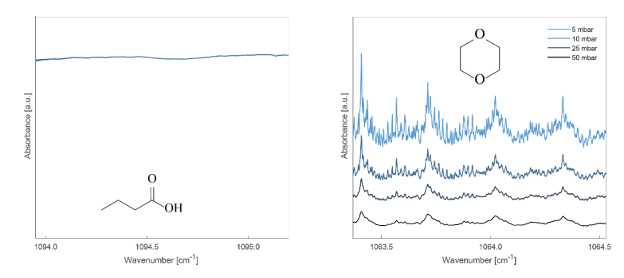
Figure 1. Absorption spectra of butyric acid (left) and 1,4-dioxane (right). 1,4-dioane has a constrained ring structure and shows distinct features that are well-resolved at reduced gas pressure (right).
Conclusion
We demonstrated that LAS can be applied for the analysis of some larger VOCs. Our findings may initiate a paradigm shift in the analysis of organic molecules by mid-IR laser spectroscopy, in particular for breath analysis.
Acknowledgements
We acknowledge support from Zürich Exhalomics [5] and Evi Diethelm-Winteler-Stiftung.
References
[1] R. Brechbühler et al. Anal. Chem. 2023, 95, 2857
[2] M. Selaković et al. Chimia 2023, 77, 785
[3] J. Wojtas et al. Opto-Electronics Rev. 2012, 20, 26.
[4] W. Vautz and M. Schmäh Int. J. Ion Mobil. Spec. 2009, 12, 139
[5] https://www.exhalomics.ch/ (Accessed: 15.01.2024)
|
Diagnosing Pulmonary Tuberculosis in Children through Breath Analysis: An Emerging Method
Ning Sun1, Carly A. Bobak3, Lesley Workman4, Lindy Bateman4, Margaretha Prins4, Cynthia Baard4, Mark P. Nicol5, Heather J. Zar4 & Jane E. Hill1,2*
1School of Biomedical Engineering, University of British Columbia, Vancouver, BC, Canada
2Department of Chemical and Biological Engineering, University of British Columbia, Vancouver, BC, Canada
3Department of Biomedical Data Science, Dartmouth College, Hanover, New Hampshire, USA
4Department of Pediatrics and Child Health, MRC Unit on Child and Adolescent Health, University of Cape Town and Red Cross War Memorial Children’s Hospital, Cape Town, South Africa
5School of Biomedical Sciences, University of Western Australia, Perth, Australia
Background
Tuberculosis (TB) poses a significant threat to children, causing an estimated one million cases and 250,000 deaths annually. Diagnosis in children may be challenging due to nonspecific clinical symptoms, inability to spontaneously produce sputum, and low bacillary burden. Only about 30% of childhood TB cases are notified and diagnosed, with approximately 50% not having bacterial confirmation. Volatile molecules (VCs) in breath reflect alterations in the body's health status resulting from the host and/or the bacterium, as well as the microbiome. Utilizing VCs found in the exhaled breath of adults with TB disease holds promise as a triage test so that it may offer a non-invasive alternative for diagnosing pulmonary TB (PTB) in children.
Aim
(A) To investigate whether a subset of molecules in the breath of children with PTB has diagnostic utility. (B) To track the breath VCs of pediatric subjects during six months of treatment.
Methods
Age- and gender-matched children were prospectively enrolled in a TB diagnostic study in Cape Town, South Africa, and breath samples were collected. The disease was categorized as confirmed PTB (microbiological diagnosis), unconfirmed (clinically diagnosed), and unlikely TB (non-TB respiratory tract illness). From April 2017 to December 2017, 31 children were enrolled in an initial cohort, with one breath sample collected before the commencement of treatment. From December 2017 to September 2023, a further 143 children were enrolled and followed. Breath samples were collected before treatment and at 1, 3, and 6 months followup. The studies followed all protocols approved by the Institutional Review Board.
Breath was collected from each child using a straw, directed into a 1L Tedlar bag, and then concentrated onto a thermal desorption (TD) tube. The TD tube was capped and stored at 4°C until analysis. Breath analysis was conducted via two-dimensional gas chromatograph tandem time of flight mass spectrometry (GC×GC-ToFMS). Chromatographic data were aligned, and volatile compounds were assigned putative names based on spectral library match using ChromaTOF software. Statistical analyses and putative biomarker discovery using machine learning tools were performed in R.
Key Results
(A) In the initial cohort (10 confirmed TB, 11 unconfirmed TB, and 10 unlikely TB with a mean age of 6.0 ± 3.1 years), a set of four breath biomarkers was evaluated in characterizing the three mentioned statuses, achieving a sensitivity of 80% and a specificity of 100% (Fig.1). (B) In the second cohort (59 confirmed TB, 57 unconfirmed TB, and 27 unlikely TB with a mean age of 7.3 ± 3.3 years), a list of 116 molecules that contributed to the alterations in breath profiles during treatment was tracked from a subset of subjects. (C) Among them, two out of four published breath biomarkers were identified, of which 4- methyl octane significantly increased after treatment. Other molecules were selected to reliably predict the treatment at 1, 3, and 6 months, respectively (Table 1).
Conclusions
A set of breath biomarkers can discriminate PTB from other lower respiratory illnesses in children. Breath biomarkers also show promise in monitoring the TB treatment response.
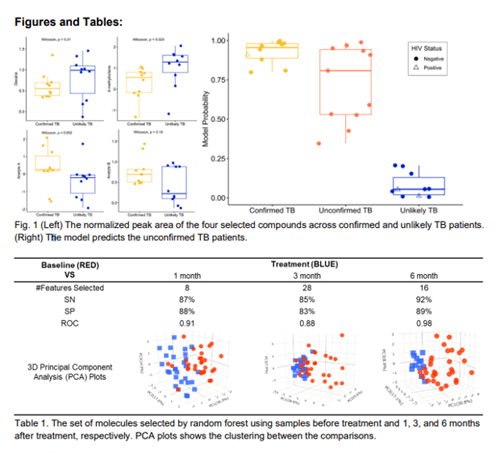
|
Canine-Inspired Identification of Volatile Organic Compound Biomarkers for Noninvasive Health Monitoring: Opportunities in the 21st Century for Biotechnology
Mark Woollam1,2* and Mangilal Agarwal1,2,3
1Integrated Nanosystems Development Institute, Indiana University Indianapolis, IN, United States.
2Chemistry and Chemical Biology, Indiana University Indianapolis, IN, United States.
3Biomedical Engineering and BioHealth Informatics, Luddy School of Informatics, Indiana University Indianapolis, IN, United States.
*Presenting author; mwoollam@iu.edu
Background
Canines have the remarkable capability of smelling volatile organic compounds (VOCs) emanating from exhaled breath and other noninvasive biofluids to distinguish diverse medical conditions. Researchers around the world for decades have been exploring the chemical composition of breath and how this information can be utilized for biomedical applications. Patients with diseases including but not limited to diabetes, COVID-19, and different types of cancer can benefit from a noninvasive means of screening and diagnosis, and VOC profiling may be a promising avenue for exploration.
Objective
This research seeks to develop analytical methods to profile VOCs in exhaled breath with high sensitivity, and to use these techniques to identify specific analytes and biomarker panels for different medical conditions including COVID-19 and hypoglycemia. Strides are also taken to develop a more fundamental understanding of the VOC profiles in healthy breath along with their cross-sectional and longitudinal reproducibility.
Methods
Approximately 3L of exhaled breath was collected through a viral filter into a Tedlar bag to identify VOC biomarker panels for COVID-19 and hypoglycemia. VOCs collected in bags were transported to the laboratory, cryothermally transferred to headspace vials loaded with glass wool, and stored at -80℃ until analysis by solid phase microextraction (SPME) coupled to gas chromatography-mass spectrometry (GC-MS). For profiling VOCs in healthy breath, a direct breath sampling method (termed DB-SPME) was developed, standardized through capnography, and optimized for sensitivity. VOCs collected on to SPME fibers for both methods were analyzed using a GC-MS quadrupole time-of-flight system. Biostatistical analysis was undertaken on the collected data to identify biomarkers through univariate and multivariate approaches.
Results
VOC results for COVID-19 revealed that subjects with a positive diagnosis had a significantly higher number of compounds and total integrated signal. Individual analytes had relatively high accuracy alone, and pattern recognition through machine learning identified a biosignature of three VOCs that could distinguish COVID-19 with over 95% accuracy. Most interestingly, samples collected from those who recovered (two months after initial diagnosis) showed that the identified breath-based biomarkers are restored to healthy baseline levels. Chemometric analyses were also employed for hypoglycemia biomarker discovery, and a separate unique panel of six VOCs was identified that could detect hypoglycemia with sensitivity = 94.8% and specificity = 95.0%. When this model was blindly tested on independently collected samples, hypoglycemia was classified with sensitivity = 90.0% and specificity = 89.9%. Analyses of breath samples from a cohort of healthy volunteers were also accomplished, which showed cross-sectional VOC measurements were significantly more variable compared to those collected longitudinally. Additionally, correlation analyses of VOCs and confounding variables showed male volunteers had significantly elevated levels of isoprene relative to women (p=0.04).
Conclusion
The presented results can ultimately inspire the design and development of an integrated nanosensor array in the form of smart and connected breathalyzers that can be used for noninvasive health monitoring rapidly at a point-of-care.
Conflict of Interest
Mangilal Agarwal has an ongoing collaboration with the NANOZ company and Scosche Industries to commercialize sensors to detect VOCs presented in this work for noninvasive health monitoring applications. All other authors report no conflicts of interest.
Ethics Board Approval
All subjects provided written consent to participate in this study, and Institutional Review Board (IRB)/Ethics Committee approval was obtained (IRB #’s 12954, 2008193976). Institutional Biosafety Committee (protocol #IN-1301) approval was also obtained at Indiana University (IU).
|
Assessment of Exhaled Breath VOC Reproducibility on Tenax®GR Thermal Desorption Tubes
Darakshan Zabin1, Trenton J. Davis1,2, Ivan V. Ivanov3, Heather D. Bean1,2
1School of Life Sciences, Arizona State University, Tempe, AZ
2Center for Fundamental and Applied Microbiomics, The Biodesign Institute, Tempe, AZ
3Department of Veterinary Physiology & Pharmacology, Texas A&M University, College Station, TX
Background
We are conducting breath volatile organic compound (VOC) studies in partnership with research teams across North America, utilizing sorbent-packed thermal desorption tubes (TDTs) for breath VOC sample concentration, storage, and transport. Most of our studies are focused on identifying the differences in breath VOC profiles between groups of subjects (e.g., biomarkers that differentiate diseased vs. healthy cohorts), but within each cohort there are several sources of breath VOC variation that impact the study, including variation between subjects in a cohort, between breath samples within subject, and between TDTs. Additionally, storage time may also interact with these sources of variation and affect breath VOC sample reproducibility.
Objectives/Aims
In this study we aimed to quantify the reproducibility of breath VOC samples on TDTs within a subject and between subjects and assess the interaction of storage time with these sources of variation.
Methods
All procedures were approved by the U.S. Army Medical Research and Development Command (USAMRDC), Office of Human and Animal Research Oversight (OHARO), and Office of Human Research Oversight (OHRO; log numbers E03915.1a and E03915.1a-1). Four subjects were recruited to provide three breath samples in 10 L Tedlar bags. All breath samples were collected on the same day, and within 120 minutes of sample collection, each sample was split into 0.6 L technical replicates by transferring onto Tenax® GR TDTs. TDTs were stored at 4 °C and technical duplicate TDTs were analyzed after 0.5 to 32 days of storage using thermal desorption – comprehensive two-dimensional gas chromatography – time of flight mass spectrometry (TD–GC´GC–TOFMS). After data processing and alignment of 152 TDT breath samples, sample reproducibility and dissimilarity were assessed using pairwise distance metrics.
Results
On average, we found that within-subject breath VOC samples were more similar than between-subject samples, and that the magnitude of variability of exhaled breath VOCs was similar across technical replicates and subjects. We also found that the storage of breath samples at 4 °C for up to one month did not affect the reproducibility of technical duplicate samples.
Conclusions
The magnitude of within-subject variation and TDT-to-TDT variation is small for breath samples collected on the same day. Further, technical replicate reproducibility is not impacted by storage times of up to 32 days.
Acknowledgements and Disclosures
This work was supported by the Defense Advanced Research Projects Agency (DARPA) Fatigue Assessment via Breath (FAB) study (Cooperative Agreement HR00112220040; Roozbeh Jafari and Linda Katehi, PIs). The views, opinions, and/or findings contained in this material are those of the authors and should not be interpreted as representing the official views or policies of the Department of Defense or the U.S. Government. No official endorsement should be inferred. There are no conflicts of interest or financial disclosures for this study.
|